Understanding Biogas Composition and Its Benefits
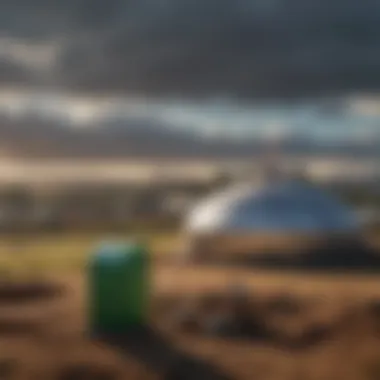
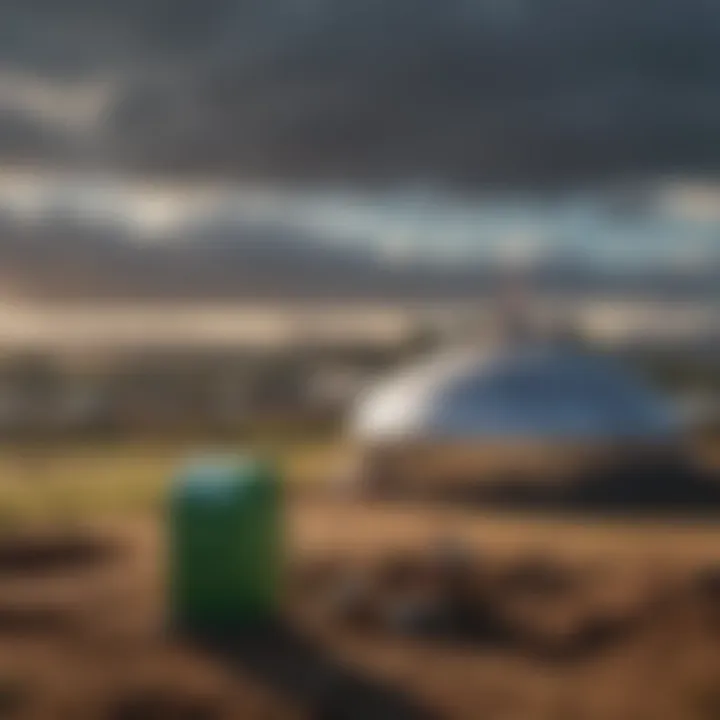
Intro
Biogas is an increasingly relevant topic in the context of sustainable agriculture and energy production. Its composition consists mainly of methane and carbon dioxide, but it also contains smaller amounts of other gases. Understanding the nuances of biogas is crucial for those interested in maximizing its benefits, particularly for farmers and agricultural enthusiasts. This article will discuss the primary components of biogas, the processes that produce it, and its implications for environmental sustainability. By delving into these aspects, the reader will gain insight into the technologies and research driving the adoption of biogas in modern practices.
Key Concepts and Terminology
Biogas is a complex mixture, and to fully grasp its implications, it is essential to understand several key concepts.
Definition of Terms
- Biogas: A mixture of gases produced through the anaerobic digestion of organic matter. The predominant gases are methane (CH₄) and carbon dioxide (CO₂).
- Anaerobic Digestion: A biological process in which microorganisms break down organic material in the absence of oxygen, resulting in the production of biogas and digestate.
- Substrates: Materials used in the production of biogas, which can include agricultural waste, animal manure, and food waste.
Overview of Relevant Practices
The production of biogas relies on specific practices that enhance its efficiency. Some common methods include:
- Proper selection of substrates to optimize biogas yield.
- Maintenance of optimal temperature and pH levels during anaerobic digestion.
- Utilization of inoculants to increase the population of methanogenic organisms.
"Biogas production is not just limited to large-scale industrial plants; it can be successfully implemented on smaller scales, benefiting local communities and farms."
Current Trends and Innovations
Biogas is evolving, with numerous research and development efforts focused on its potential.
Latest Research and Developments
Research is expanding the knowledge of how to maximize biogas production. Studies often examine:
- The impacts of different substrates on gas yield.
- Advanced anaerobic digestion techniques.
- Integration of biogas systems with other renewable energy sources.
Emerging Technologies and Tools
Technological advancements are playing a crucial role in the biogas sector. Notable innovations include:
- Biogas purification systems: These technologies enhance the quality of biogas, making it more suitable for commercial applications.
- Smart monitoring systems: These allow real-time analysis of the anaerobic digestion process, leading to better management decisions.
Practical Applications
Understanding how to leverage biogas can greatly benefit farmers and agricultural enthusiasts.
Step-by-Step Guides
- Assess Available Resources: Identify the types of organic waste available for digestion.
- Choose a Digestion Method: Decide between continuous flow or batch digestion based on scale and resources.
- Monitor Conditions: Regularly check the pH and temperature to ensure optimal digestion.
- Utilize Byproducts: Implement methods to use digestate as a fertilizer, enhancing soil health.
Troubleshooting Common Issues
Common challenges in biogas production can hinder progress. Some solutions include:
- Fluctuating Gas Yield: Monitor substrate ratios to identify any imbalance.
- Odor Issues: Ensure proper mixing and processing of the feedstock to reduce foul odors.
This comprehensive exploration of biogas composition lays a foundation for understanding its benefits and challenges in sustainable practices. The insights gained here can serve as a guide for more effective implementation in agricultural settings.
Intro to Biogas
Understanding biogas is crucial in today's society as it stands at the intersection of agricultural innovation and renewable energy. As the world faces increasing environmental challenges, the need for sustainable solutions becomes more pressing. Biogas, a product obtained from the anaerobic degradation of organic materials, presents a dual opportunity: reducing waste and providing a renewable energy source.
Biogas is not merely an energy source; it has profound implications on sustainable agriculture and waste management. By utilizing agricultural waste, livestock manure, and other organic materials, biogas systems can effectively contribute to waste reduction. This has implications for farmers and agricultural enthusiasts as it promotes a circular economy and sustainability in agricultural practices.
Definition of Biogas
Biogas is a type of biofuel that is produced through the anaerobic digestion of organic matter. This process occurs in the absence of oxygen and involves a complex interplay of microorganisms that break down biodegradable materials. The primary components of biogas include methane, carbon dioxide, nitrogen, and trace gases. Methane, often referred to as biomethane, is the main energy-rich component, making up about 50% to 70% of the total composition, while carbon dioxide accounts for roughly 30% to 50%.
The presence of methane makes biogas a potent source of energy, enabling its use in various applications, ranging from electricity generation to heating and fueling vehicles. Its renewable nature is derived from the fact that it is sourced from organic waste products, thus representing a sustainable alternative to fossil fuels.
Importance of Biogas in Sustainable Agriculture
Biogas holds significant importance in the sphere of sustainable agriculture. Firstly, its production reduces the burden of waste generated from farms. Waste materials, such as animal manure and crop residues, can lead to environmental pollution if not managed properly. Converting these materials into biogas mitigates such risks and transforms potential pollutants into valuable energy.
Moreover, the use of biogas in agricultural practices contributes to energy self-sufficiency for farmers. By harnessing locally available organic materials, farmers can generate their own energy, reducing dependency on external energy sources. This aspect can improve economic viability and resilience against fluctuating energy prices.
Furthermore, the by-product of the biogas production process, commonly known as digestate, is rich in nutrients. Farmers can utilize this organic fertilizer to enhance soil quality, promote healthier crop growth, and reduce the need for chemical fertilizers, which can be harmful to the environment.
In summary, biogas not only enables the harnessing of renewable energy but also fosters efficient waste management, nutrient recycling, and resilience within agricultural systems. The integration of biogas production in agriculture aligns with the principles of sustainability and provides a pathway towards more eco-friendly farming practices.
Primary Components of Biogas
Biogas is primarily made up of gases produced during the anaerobic digestion process. Recognizing the components of biogas is crucial to understanding its value and applications in agriculture and energy production. The composition impacts its energy content, usability, and environmental implications. Biogas mainly consists of methane, carbon dioxide, and trace gases, which together give insights into its potential as a renewable energy source.
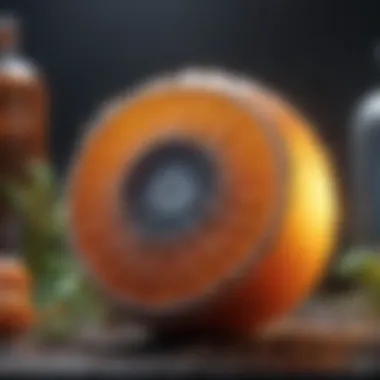
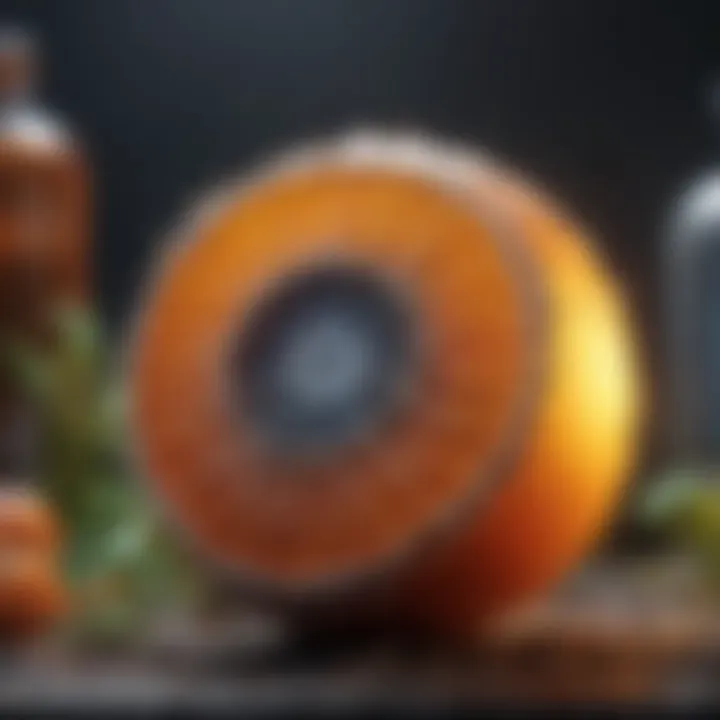
Methane: The Key Component
Methane is the most significant component of biogas, typically comprising 50% to 70% of the total gas produced. It is highly flammable and can be used for various applications such as heating, electricity generation, and as a vehicle fuel. The presence of methane is vital for the energy efficiency of biogas systems. By utilizing methane, farmers can reduce their dependency on fossil fuels, which contributes to sustainability in agriculture.
The high energy content of methane, ranging from 35 to 40 megajoules per cubic meter, makes it the focus of biogas research. Understanding how to enhance methane production is critical for improving the overall efficiency of the biogas systems.
Carbon Dioxide and Its Role
Carbon dioxide accounts for about 30% to 50% of biogas composition. While it does not possess direct energy value like methane, it plays an important role in various contexts. For example, the presence of carbon dioxide in biogas can affect its quality and the efficiency of energy production after combustion. Furthermore, carbon dioxide can contribute to the greenhouse gas emissions if released into the atmosphere without capture.
Therefore, capturing and utilizing carbon dioxide is essential in advancing sustainable practices. Some innovative applications include using carbon dioxide in greenhouses to enhance plant growth or converting it into carbonates for other industrial uses.
Trace Gases in Biogas
Trace gases, while present in smaller quantities, can significantly affect the performance and safety of biogas applications. Important trace gases include hydrogen sulfide, ammonia, and water vapor.
Hydrogen Sulfide
Hydrogen sulfide is usually present in biogas at trace levels, typically between 0.5% and 3%. It is a relevant byproduct of the anaerobic digestion of organic materials. The primary concern with hydrogen sulfide is its toxicity and corrosive properties. Although it is not beneficial in higher concentrations, some argue that small amounts can be used in certain chemical processes.
The main characteristic of hydrogen sulfide is its strong odor, often described as having a ‘rotten egg’ smell. This quality serves as a warning sign for detection, highlighting the need for safety measures when handling biogas. Its removal from the biogas stream is essential before energy conversion processes to avoid equipment damage.
Ammonia
Ammonia can also be found in biogas, ranging from 0.1% to 0.5%. It is produced during the breakdown of nitrogen-containing organic materials. Ammonia has significant implications for nutrient management in agriculture, as it can be reused as fertilizer. This characteristic aligns with circular economy principles by recycling waste into valuable products.
However, excessive ammonia can inhibit microbial activity in anaerobic digestion, affecting overall gas production. Therefore, monitoring and controlling ammonia concentrations is necessary to maintain optimal performance of biogas systems.
Water Vapor
Water vapor is consistently present in biogas, its concentration typically between 0.5% and 10% by volume. While it does not contribute to energy production, its presence can impact the efficiency of biogas utilization. High levels of water vapor can lead to condensation in storage and transport systems, causing operational issues.
Managing water vapor is essential for ensuring gas quality and preventing equipment failures. In summary, while water vapor adds complexity to handling biogas, it also provides insight into moisture content of substrates and potential operational adjustments.
Understanding these trace gasses enhances the knowledge of biogas properties and helps to navigate safety and operational challenges.
Biogas Production Process
The biogas production process is a critical element in understanding how biogas is generated and its potential applications in agriculture. This process involves a series of biological and chemical reactions that convert organic materials into biogas. Understanding this process provides insights into optimizing biogas production and managing the feedstock effectively.
Anaerobic digestion is the main process through which biogas is produced. In this process, microorganisms break down organic materials in the absence of oxygen, resulting in the formation of biogas. The efficiency of this process can vary significantly based on several influencing factors. For example, temperature, pH levels, and retention time are all crucial parameters that can enhance or hinder biogas output. By comprehending these factors, farmers can tailor their biogas systems to maximize productivity.
Furthermore, the knowledge of the microorganisms involved is essential. Both bacteria and archaea play vital roles in the digestion process. They contribute to the breakdown of complex organic compounds into simpler ones, ultimately leading to the generation of methane and carbon dioxide. This understanding allows operators to manage conditions better in their anaerobic digesters for optimal microbial activity. Hence, the biogas production process is not just about the biological transformation; it also involves strategic management to harness its full potential for agricultural benefits.
Anaerobic Digestion Explained
Anaerobic digestion is the heart of biogas production. It involves several stages, each performed by different microorganisms and operating under specific conditions. The process starts with hydrolysis, where complex organic matter is broken down into soluble sugars and amino acids. Next, acidogenesis occurs, turning these products into volatile fatty acids, alcohols, and hydrogen.
Finally, in the methanogenesis stage, methanogenic archaea convert these simpler compounds into methane and carbon dioxide. The resulting biogas is typically composed of around 60% methane, 30% carbon dioxide, and trace gases. Enhancing the anaerobic digestion process can substantially increase biogas yields.
Microorganisms Involved in Biogas Generation
Bacteria
Bacteria are critical for the anaerobic digestion process. They initiate the breakdown of organic matter and convert it through different stages. The specific aspect of bacteria that is essential is their diversity. Various bacteria species target different substrates and transform them into simpler compounds. This adaptation allows for the effective utilization of a wide range of organic materials, making bacteria a universal choice for biogas production.
One key characteristic of bacteria is their rapid growth rate. This feature allows them to effectively respond to varying substrate types and digestion conditions. However, bacteria also have their unique disadvantages. Some species may require specific conditions to thrive, and failure to maintain these can lead to reduced biogas yields.
Archaea
Archaea are another essential group of microorganisms in biogas production. Their role primarily revolves around methanogenesis, where they convert substrates into methane. The high tolerance of archaea to conditions that inhibit bacteria makes them a beneficial choice for biogas systems. They can thrive in high-temperature environments and under low pH levels, contributing to robustness and efficiency in digestion processes.
A unique feature of archaea is their ability to use hydrogen and carbon dioxide to produce methane. However, the slow growth rate of archaea presents a disadvantage. This characteristic can limit their recovery speed under changing conditions, possibly leading to fluctuating biogas production rates when environmental factors are not managed carefully.
Influencing Factors in Biogas Production
Temperature
Temperature plays a crucial role in the efficiency of anaerobic digestion. Microbial activity increases with temperature, leading to higher biogas production rates. Generally, there are two temperature ranges utilized: mesophilic (about 30-40°C) and thermophilic (50-60°C). Mesophilic digestion is often favored for its stability, while thermophilic digestion can yield biogas quicker.
However, maintaining optimal temperatures can be challenging and requires substantial energy input. If the temperature fluctuates too much, it can inhibit microbial activity, thus affecting the amount of biogas produced.
pH Level
The pH level is another significant influencing factor in biogas production. Most bacteria and archaea prefer a neutral pH range of 6.5 to 7.5. If the pH falls outside this range, it can create an environment that is unsuitable for microbial activity. Too high or too low pH levels can lead to reduced biogas yields and even process failure.
Regulating the pH requires careful management of the feedstock and may need adjustments using different additives, which can add to operational costs. Nevertheless, achieving the right pH balance is essential for optimizing overall biogas production.
Retention Time
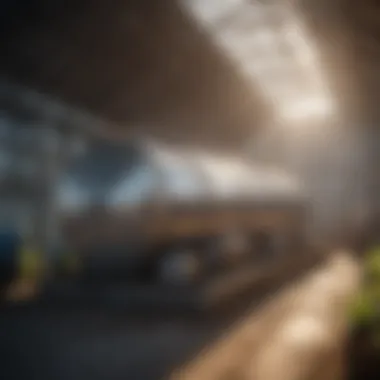
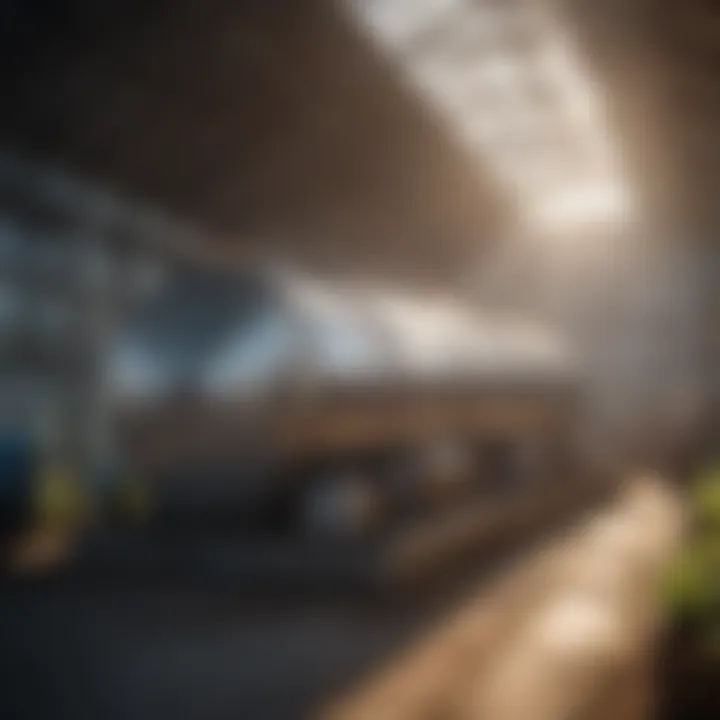
Retention time refers to the duration that the feedstock remains in the digester. Longer retention times generally provide microbes with more opportunities to break down organic matter effectively, leading to increased biogas production. However, increasing retention time may also result in higher costs related to management and space in the digestion system.
The unique balance between efficiency and economic viability is crucial. Finding the optimal retention time requires understanding the specific feedstock and desired biogas quantity. Adjustments to retention time can significantly influence the biogas generation process.
Understanding these elements is key to improving biogas systems and maximizing their impact in sustainable agriculture.
Substrates Used for Biogas Production
Substrates used for biogas production are critical. They determine both the quality and quantity of the biogas generated. Choosing the right feedstock can enhance efficiency and effectiveness in biogas systems. The correct substrates not only boost methane production but also facilitate a smoother anaerobic digestion process. Understanding the types of materials suitable for biogas is essential for optimizing the operation.
Organic Materials as Feedstock
Organic materials serve as primary feedstock for biogas production. They are biodegradable, making them ideal candidates for anaerobic digestion. This feedstock includes various types of plant and animal matter. Examples are food scraps, crop residues, and manure. Their abundance, especially in agricultural settings, reinforces their value. Moreover, using these materials helps in waste management, thus reducing environmental pollution.
Waste Materials and Their Potential
Waste materials hold significant promise for biogas generation. Their potential is often underestimated.
Food Waste
Food waste is abundant and often discarded. This squandered material can generate a high methane yield due to its rich organic composition. A key characteristic of food waste is its moisture content, which promotes anaerobic digestion. Its use is beneficial because it reduces landfill waste, contributing to sustainability. The unique feature of food waste is the diverse range of organic materials it contains, which aids in effective digestion processes. However, the disadvantage lies in contamination; proper separation from non-biodegradable items is critical to maximize its effectiveness.
Agricultural Residues
Agricultural residues are byproducts from farming activities. They include straw, husks, and other leftover plant materials. These residues present a crucial opportunity for biogas production as they are plentiful in rural areas. Their key characteristic is the high carbon-to-nitrogen ratio, important for microbial growth during digestion. Agricultural residues are popular because they offer a cost-effective solution for biogas feedstock. However, their high lignin content can make digestion more challenging, sometimes leading to lower gas yields if not pre-treated adequately.
Manure
Manure, primarily from livestock, is another valuable substrate. It is high in nutrients and has a balanced composition that supports microbial activity. The key characteristic of manure is its consistency and availability on farms. Using manure for biogas production is beneficial as it recycles waste from livestock farming while producing energy. One unique feature is its nutrient richness, which also benefits the digestate used as fertilizer. The downside to using manure can be its handling and storage requirements, which can complicate the logistics of biogas systems.
Comparative Analysis of Substrate Types
A comparative analysis of different substrate types is important for maximizing biogas production. Each material has unique properties that influence efficiency. For example:
- Food waste: High moisture, rich in carbohydrates
- Agricultural residues: Abundant, but may require pretreatments
- Manure: Nutrient-rich, easy to obtain but may need careful handling
By analyzing these factors, stakeholders can make informed decisions, choosing substrates that best fit their operational needs and environmental goals. This can lead to improved biogas yields and sustainable agricultural practices.
Applications of Biogas in Agriculture
Biogas has emerged as a pivotal element in modern agricultural practices. Its applications extend beyond mere energy production, influencing various dimensions of farming and sustainability. The use of biogas offers substantial benefits in terms of renewable energy sources while simultaneously reducing waste. This section will delve into two principal applications of biogas in agriculture: its function as a renewable energy source and the potential to produce organic fertilizer from digested sludge.
Use as Renewable Energy Source
Biogas serves as a crucial renewable energy source, especially in rural areas where fossil fuel access might be limited or economically unfeasible. Derived from organic materials through anaerobic digestion, biogas primarily comprises methane and carbon dioxide, with methane being the valuable component that allows for energy generation.
Benefits of using biogas as an energy source include:
- Cost-Effectiveness: Farmers can utilize biogas to generate electricity and heat, which minimizes reliance on external energy supplies and reduces costs.
- Energy Independence: By harnessing biogas, farms can achieve greater energy autonomy, thus lessening their vulnerability to fluctuations in energy prices.
- Environmental Benefits: Utilizing biogas for energy reduces greenhouse gas emissions compared to conventional fossil fuels, thereby contributing to climate change mitigation.
Moreover, the surplus energy produced can be fed back into the grid or used to power farm equipment, accelerating sustainable farming practices while generating additional revenue streams.
Organic Fertilizer from Digested Sludge
The digestate, a by-product of biogas production, has significant potential as an organic fertilizer. After anaerobic digestion, the leftover material is rich in nutrients necessary for plant growth. This offers an effective alternative to chemical fertilizers that often carry environmental implications.
Key advantages of using digested sludge as fertilizer include:
- Nutrient-Rich Composition: The digestate contains essential nutrients such as nitrogen, phosphorus, and potassium, fostering healthy soil and optimal crop growth.
- Soil Health Improvement: Regular application of organic fertilizers contributes to enhanced soil structure, promoting better water retention and microbial activity.
- Waste Reduction: Utilization of digestate tackles waste management issues while transforming agricultural residues into valuable fertilizers, thereby closing nutrient loops on farms.
"The transformation of waste into wealth is a compelling narrative of sustainability in agriculture, embodying the concepts of circular economy."
Environmental Impact of Biogas
The environmental impact of biogas is multifaceted, addressing both the benefits and challenges associated with its use in agriculture and energy generation. Understanding these implications is essential for farmers and enthusiasts who are keen on adopting sustainable practices in their operations. By effectively capturing and utilizing biogas, we can mitigate many environmental concerns that arise from traditional waste management practices.
Reducing Greenhouse Gas Emissions
One of the most significant benefits of biogas utilization lies in its potential to reduce greenhouse gas emissions. Methane is a potent greenhouse gas, with a global warming potential much greater than carbon dioxide over a century. However, when organic materials decompose anaerobically, they can release methane into the atmosphere if not properly managed. Biogas systems capture this methane and convert it to energy, thus preventing it from contributing to global warming.
- By capturing methane via anaerobic digestion, biogas reduces emissions by:
- Furthermore, using biogas as an energy source replaces fossil fuels, offering lower emissions compared to traditional energy generation.
- Decreasing methane release from landfills.
- Preventing methane escape from manure management.
Research shows that biogas systems can lead to reductions of over 50% in net greenhouse gas emissions compared to conventional waste disposal methods and fossil fuel usage. This reduction plays a vital role in addressing climate change.
Sustainable Waste Management Practices
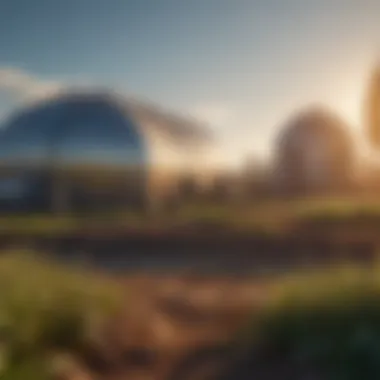
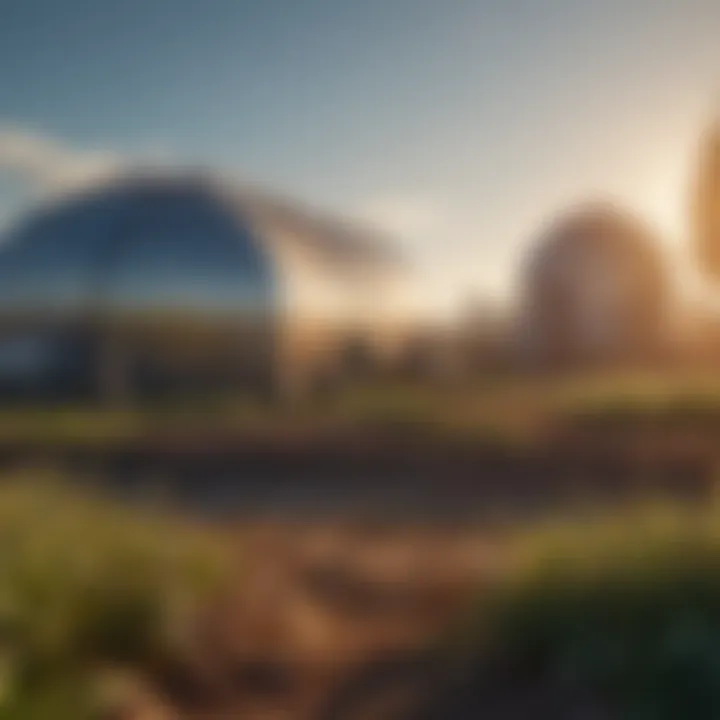
Biogas production also contributes to sustainable waste management practices. As waste accumulation becomes increasingly problematic, especially in urban areas, the need for effective waste treatment is crucial.
- Utilizing biogas systems can:
- Divert organic waste from landfills, significantly reducing landfill volume.
- Minimize odors and leachates that often accompany decomposition in landfills.
- Enhance soil health by producing digestate, a nutrient-rich organic fertilizer.
Moreover, the capture of biogas can be effectively integrated into waste treatment facilities. This integration not only ensures that waste is managed sustainably but also produces a renewable energy source, fostering a circular economy mindset.
"Biogas systems transform challenges in waste management into opportunities for both environmental protection and energy generation."
Through these practices, biogas serves as a cornerstone for sustainable agricultural and environmental management, presenting a clear pathway towards more responsible stewardship of our natural resources.
Challenges in Biogas Adoption
The adoption of biogas technology in agriculture faces a number of challenges that must be addressed for its successful implementation. Understanding these challenges is crucial for farmers and stakeholders aiming to optimize the benefits of biogas. Each issue presents unique aspects that affect overall efficiency and growth of biogas use. Given that sustainable farming practices increasingly incorporate biogas systems, analyzing economic, technological, and regulatory barriers helps in creating frameworks for effective solutions.
Economic Barriers
Economic barriers can significantly hinder the widespread acceptance of biogas technology. Initial capital investment for biogas plants is substantial, which can deter farmers who are uncertain about the return on investment. Additionally, operational costs such as maintenance and staffing might be prohibitive for small-scale farms. Without sufficient financial support or incentives from governments or institutions, many farmers may not see biogas as a viable option.
Moreover, fluctuating prices of fossil fuels can impact the economic viability of biogas. When traditional energy sources are cheaper, the incentive to invest in renewable energy diminishes. The lack of stable and favorable market conditions can make it difficult for farmers to justify the switch to biogas.
Technological Limitations
Technological limitations also pose challenges for biogas adoption. Not every farm has access to the necessary technology or expertise to operate a biogas plant effectively. Advanced biogas technologies require skilled labor for construction, operation, and management, which may not be readily available in all regions.
In addition, the efficiency of biogas production depends heavily on the quality of feedstock and process management. Simple systems may not yield enough gas to cover operational costs. This underperformance can deter farmers who seek guarantees on their investments. As a result, there is a pressing need for research and development in biotechnology to create more efficient, user-friendly systems that cater to diverse agricultural contexts.
Policy and Regulation Issues
Policy and regulation issues further complicate biogas adoption. In many countries, the regulatory framework surrounding renewable energy is still developing. Farmers often encounter a lack of clarity on the rules governing biogas plants. This ambiguity can result in delays in project approvals or increase compliance costs.
Additionally, policies that favor traditional fossil fuels over renewables create an uneven playing field. Without supportive frameworks, such as subsidies or feed-in tariffs, it is difficult for biogas initiatives to gain momentum.
"Supportive policies are critical to ensuring the long-term success of biogas systems in agriculture. Without them, many viable projects may not materialize."
In summary, addressing the economic barriers, technological limitations, and regulatory issues is essential for advancing biogas adoption in agriculture. Incorporating these considerations into broader strategies can enhance the feasibility of biogas systems and promote sustainable agricultural practices.
Future of Biogas in Agriculture
The future of biogas in agriculture is pivotal. This renewable energy source has the potential to significantly enhance farming practices and sustainability. As the world faces challenges from climate change and waste management, biogas emerges as a viable solution. It not only provides energy but also supports soil health through the use of digestate as fertilizer. Such benefits are crucial for modern agriculture, where maximizing outputs while minimizing environmental footprints is increasingly important.
Technological Innovations and Their Impact
Technological advancements are driving the evolution of biogas production. Innovations in anaerobic digestion processes are improving efficiency and output. For instance, developments in microbial fuel cell technology can optimize the breakdown of organic materials. This allows for greater methane capture and reduces waste. Furthermore, the integration of automation and monitoring systems enhances process control, leading to more consistent biogas production.
Another area of innovation is in substrate processing. Advances like pre-treatment processes help in breaking down complex organic materials, making them more accessible for decomposition. These technologies can expand the range of substrates, enabling the use of diverse agricultural residues and waste products.
Additionally, research into genetically engineered microorganisms aims to increase the overall yield of biogas. These developments can lead to a more robust system capable of handling variations in feedstock composition. Overall, these innovations are paving the way for a sustainable and efficient biogas industry, crucial for agricultural advancement.
Policy Trends Supporting Biogas
Policy frameworks play a vital role in promoting biogas use in agriculture. Governments are recognizing the importance of renewable energy sources in achieving sustainability goals. Many countries are implementing incentives for biogas projects, such as grants and favorable tax rates. These incentives make investments in biogas more attractive for farmers and entrepreneurs.
Additionally, regulations aimed at reducing greenhouse gas emissions foster biogas adoption. By setting ambitious targets for emission reductions, governments encourage the transition towards cleaner energy options. Notably, policies that support research and development in biogas technologies can significantly enhance innovation and market growth.
Public awareness campaigns also contribute to the favorable policy climate. By highlighting biogas’s environmental benefits, such campaigns create a supportive atmosphere for legislation. Collaboration with agricultural organizations further reinforces these efforts, ensuring that farmers are aware of the potential advantages of biogas.
"The integration of biogas technology into agriculture not only supports energy needs but also enhances soil fertility, representing a unique convergence of environmental stewardship and economic viability."
In summary, the future of biogas in agriculture hinges on technological innovation and supportive policies. Together, these elements can drive the transition towards a resource-efficient agricultural system, benefiting both farmers and the environment.
Culmination and Key Takeaways
In examining the composition of biogas, it is clear that this renewable energy source offers a range of environmental and agricultural benefits. Biogas is primarily composed of methane and carbon dioxide, with minor constituents such as hydrogen sulfide and ammonia. Understanding these components not only sheds light on the efficacy of biogas as a sustainable energy source but also its impact on reducing greenhouse gas emissions.
Factors influencing biogas production, including technology and substrate selection, play a significant role in maximizing its potential. For farmers and agricultural enthusiasts, the insights gained from this article can guide effective practices that enhance farm sustainability and resource management.
- Biogas as a Renewable Energy: The ability to utilize biogas for energy needs creates a circular economy on farms, reducing waste while providing energy.
- Nutrient-Rich Fertilizer: The byproducts of biogas production, such as digestate, serve as organic fertilizers that improve soil health.
- Environmental Benefits: By mitigating methane emissions from waste, biogas contributes to climate change mitigation.
This holistic understanding of biogas highlights its essential role in supporting sustainable agriculture. More efforts to educate farmers about biogas production can significantly enhance its adoption, leading to greater sustainability in farming practices.
Summary of Biogas Composition and Applications
Biogas is primarily composed of about 50-70% methane, which is the fuel component, and 30-50% carbon dioxide. The trace gases, such as hydrogen sulfide, can pose challenges in biogas management but are manageable with proper filtration techniques. These gases interplay in the anaerobic digestion process, creating an effective method for waste treatment and energy generation.
Applications of biogas extend into various aspects of agriculture. Its use as a renewable energy source reduces dependence on fossil fuels, while its byproducts can enhance soil fertility when used as fertilizers. This blend of energy and nutrient supply illustrates the versatility of biogas.
Call for Increased Research and Development
There is a pressing need for increased research and development in biogas technology. Innovations can enhance the efficiency of biogas production, making it a more viable option for farmers. Studies focusing on optimizing fermentation processes, improving metabolic pathways, and developing cost-effective systems are crucial for the scalability of biogas adoption in agriculture.
Furthermore, collaboration between universities, agricultural organizations, and governmental bodies could lead to more effective policies and regulations that support biogas initiatives.
By prioritizing research and innovation, we can unlock the full potential of biogas as a significant player in sustainable agriculture and energy production, benefitting both the environment and the agricultural community.